This page will list projects completed during my Polonez fellowship.
Magneto-plastic dynamics of a highly-stressed neutron-star crust
X-ray bursts and gamma-ray flares from highly magnetised neutron stars rank among the most violent, energetic phenomena observed by astronomers. It is thought that the released energy from this activity was originally built up as stresses in the star’s crust as its magnetic field evolved. The neutron-star crust, like any elastic material, has a yield point – so, once the stresses get too high, the crust breaks. Or more accurately, it starts to deform plastically.
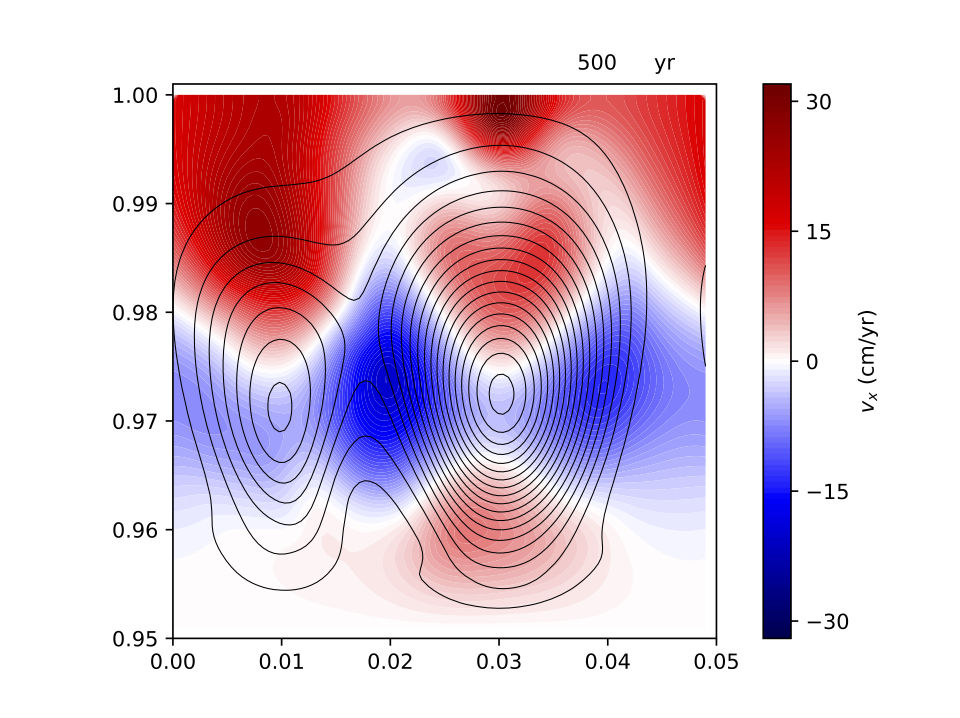
For the first time in a quantitative model of the crust’s magnetic-field evolution, we were able to track the build-up of stresses, and the point at which the crust reached its yield point. After that, we solved for the way in which the magnetic field and the plastic flow evolve together. We found that the plastic motions at the surface are relatively fast – tens or hundreds of centimetres per year – and these would indeed be likely to induce flares and bursts outside the star.
The final paper may be found here, or in preprint form here.
Evolution of a neutron star’s inclination angle
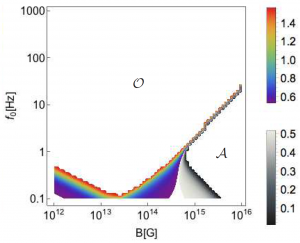
Two of the most fundamental attributes of a neutron star – indeed, any star – are its rotation and its magnetic field. The axis about which a neutron star rotates is generally not the same as the symmetry axis for its magnetic field though; they are misaligned by some angle χ. Observationally, there are suggestions that: this angle decreases over million-year timescales, that the distribution is bimodal (with χ close to either zero or ninety degrees), and that the value of χ for the famous Crab pulsar may have increased by an observable amount since we first started observing it a few decades ago. Can all these observations be reconciled with one model? Do they make sense on theory grounds?
It has been known since the 1970s that there are two competing physical mechanisms for the evolution of χ in a star; one acts to bring the two axes into alignment, and the other acts to orthogonalise them. We recently managed to assemble all the physical ingredients needed to study this problem quantitatively for the first time and showed how the final value of χ for a highly-magnetised neutron star is probably fixed shortly after its birth, since the evolution of the angle is very quick. Many such stars will end up with almost-aligned rotation and magnetic axes.
The final paper may be found here, or in preprint form here.
Heat conduction in rotating relativistic stars
A quantitative understanding of the cooling of a young neutron star needs to allow for general relativity, and the star’s rotation. The equation which governs heat conduction for a star in general relativity looks virtually the same as its Newtonian equivalent, but both are parabolic: they predict that if the local temperature changes, that information is known immediately everywhere else. This would violate a basic principle of relativity, called causality: that nothing travels faster than light.
Fortunately, we were able to show that by starting from a more general theory – which does satisfy causality – one can indeed derive the heat equation in its familiar form. The equation would have causal terms, but one can often assume that they act over such short timescales that their contribution is negligible. Rotation terms are also absent from the equation, unless the star rotates very fast. So, we got back to the expected form of the heat equation, but with an understanding of how and when this equation will cease to be valid (in more dynamical processes like the inspiral of two neutron stars, for example).
The final paper may be found here, or in preprint form here.